Introduction
The anthropogenic increase in atmospheric CO2 concentration is a major environmental concern. One alarming consequence is a rapid change in seawater chemistry and decrease of ocean pH (Solomon et al. 2007; Doney et al. 2009), which could have large impacts on marine ecosystems, and pose a threat to marine life (Kerr 2010; Pelejero et al. 2010). Current evidence suggests a range of biological effects in marine organisms, but also considerable variation in the sensitivity to changes in oceanic CO2 between and within major taxa (Doney et al. 2009; Kroeker et al. 2010). The variable responses among species and taxa are suggestive of ecosystem effects, but we are currently far from being able to predict how increased CO2 affects marine communities (Kerr 2010). It is pivotal to increase our understanding of how parameters related to the fitness of organisms are affected by the on-going ocean acidification.
While it is known that increased CO2 has negative consequences for many calcifying organisms (Hoegh-Guldberg et al. 2007), effects on fish have been less clear and until recently little studied (Ishimatsu et al. 2008). Compared to invertebrates, fishes have been hypothesized to be more physiologically tolerant to elevated CO2 (P€ortner et al. 2004; Melzner et al. 2009). Despite this, there is now evidence of negative fitness consequences of near-future CO2 levels on fishes (Munday et al. 2010). Early life history stages seem especially vulnerable to environmental change, including increased CO2 levels (Baumann et al. 2012). However, evidence for direct effects of near-future CO2 levels on early developmental stages in fish is mixed (Munday et al. 2009a, 2011; Baumann et al. 2012; Frommel et al. 2012). Interestingly, effects on behaviors, such as homing, boldness, activity, and predator avoidance, have been found in coral reef fishes (Munday et al. 2009b, 2010; Dixson et al. 2010; Ferrari et al. 2011, 2012; Simpson et al. 2011), with a resulting higher mortality (Munday et al. 2010; Ferrari et al. 2011). A recent study on adult sticklebacks suggests that also behavior of temperate fishes can be affected (Jutfelt et al. 2013). These changes in behavior can largely be explained by elevated CO2 impairing sensory function (Munday et al. 2009b, 2012; Dixson et al. 2010; Simpson et al. 2011). One suggested underlying mechanism, which has received experimental support, is that CO2 interferes with brain neurotransmitter function of the GABA-A receptor, leading to neuronal overactivity (Nilsson et al. 2012). Because the GABA-A receptor is the main inhibitory neurotransmitter receptor in the vertebrate brain, increased oceanic CO2 levels may lead to multisensory and behavioral impairment in a wide range of fishes (Nilsson et al. 2012).
The scope of this study was to test effects of elevated CO2 on fitness-related parameters of a temperate, coastal fish. Such fishes live in habitats with considerable shortterm natural variation in pH, in contrast to the open ocean habitat (Hofmann et al. 2011), and may thus be expected to show some tolerance to varying CO2 levels (Melzner et al. 2009). Most CO2 experiments on marine organisms to date have focused on a single life history stage (Dupont et al. 2012). Here, we tested effects of elevated CO2 on successive life history stages (from mating to early larval performance) in an ecologically important fish species in European coastal environments, the twospotted goby (Gobiusculus flavescens). Early life stages are particularly sensitive to environmental stress in teleosts (Brown and Sadler 1989; Kikkawa et al. 2003). We therefore predicted CO2 to negatively affect egg survival or embryo development in our experiment. From the hypothesis that exposure to increased CO2 levels have effects on brain function and sensory responses (reviewed in Munday et al. 2012), we predicted an effect on behavior via the visual sensory channel. Specifically, based on Nilsson et al. (2012) finding that high CO2 causes neuronal over activity, we hypothesized that elevated CO2 would lead to sensory hypersensitivity. We therefore predicted increased positive phototaxis as a response to elevated CO2.
Results
Spawning and embryonic development
Initial clutch size (only single female spawnings included; mean ± SD) did not differ between treatments (control: 1392 ± 302 eggs, CO2: 1434 ± 255 eggs; t = 0.33, df = 18, P = 0.42). Egg incubation time varied from 9.5 to 12.5 days, but with no detectable effect of treatment (control: 11.2 ± 0.9 days, CO2: 11.4 ± 0.7 days; t = 0.39, df = 22, P = 0.70). Clutch size at day 9, was also relatively similar between treatments (control: 1556 ± 600 eggs, CO2: 1410 ± 594 eggs; t = -0.60, df = 23, P = 0.55). The proportion of eggs lost before hatching (i.e., loss of eggs between spawning and day 9) was, however, more than twice as high in the elevated CO2 treatment (14.5 ± 13.3 per cent) as in the control (6.4 ± 5.4 per cent; Mann–Whitney U test, P = 0.025). Even if most eggs and embryos developed normally (Fig. 2), inspection for abnormal embryos at high magnification (day 7) showed several instances of abnormalities, which mainly were caused by arrested development at various embryonic stages (Fig. 3). Abnormal embryonic development occurred more than twice as frequently in the elevated CO2 (6.1 ± 6.7 per cent) as in the control (2.4 ± 2.7 per cent) treatment (t = 2.09, df = 23, P = 0.048) (Fig. 3).
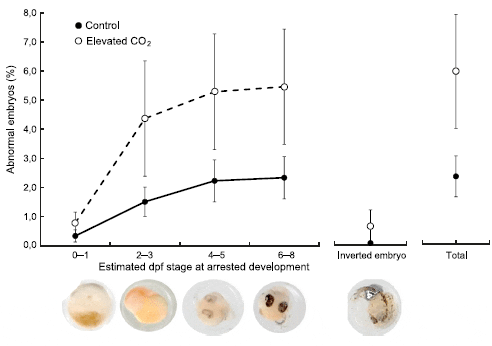
Larval phototaxis
A high proportion of the larvae showed a strong directional phototactic response, and swam quickly toward the light (mean time to swim 50 cm: 52 ± 22 sec [N = 14]; fastest larva: 13 sec). Calculated in terms of body lengths (mean SL at hatching 2.8 mm; Svensson 2006), the mean speed was 3.4 BL s-1, and 13.7 BL s-1 for the fastest larvae. Hence, the phototactic response of the larvae was very strong.
Larvae reared under elevated CO2 conditions showed a much stronger phototactic response than those reared under control conditions. In the test apparatus, 62 ± 22 per cent of larvae from the elevated CO2 treatment reached the light source within 2 min, as compared to only 36 ± 22 per cent of the control larvae (t = 2.99, df = 23, P = 0.007) (Fig. 4). The time used by the fastest larva of each clutch to reach the light also revealed a marked difference between treatments. On average, the fastest larvae from the elevated CO2 clutches used 40 ± 14 sec to reach the light, compared to 64 ± 22 sec for control treatment larvae (t = 2.47, df = 12, P = 0.029) (Fig. 4).
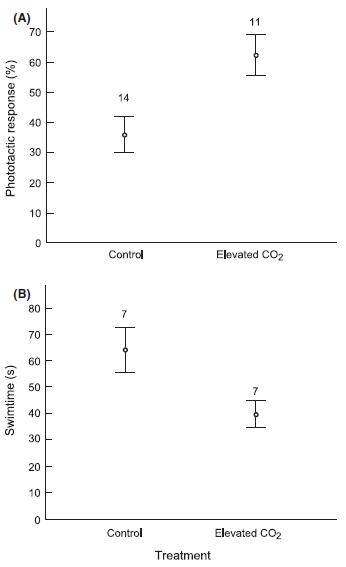
Acute effect?
There was no evidence of an acute effect of pCO2, as neither the overall phototactic response (percent reaching the light source; elevated CO2: 35 ± 14 per cent, control 41 ± 23 per cent, t = -0.75, df = 22, P = 0.46) nor the swimming speed of the fastest larva (elevated CO2: 69 ± 23 sec, control 71 ± 26 sec, t = 0.14, df = 22, P = 0.89) differed between elevated CO2 and control test environments. For both test environments, the levels were as expected for a clutch that had developed in control CO2 conditions (see Fig. 4). Hence, current test conditions (pCO2 in the swim channel) did not affect the results.
General activity
Under uniform overhead light, activity was low and not different between rearing treatments (ca 20 per cent leaving the start compartment, P > 0.8 between treatments). Activity in the dark was very low. In only one case did a larva move into the neighboring chamber (0.4 per cent of larvae). Hence, the larvae showed minimal activity in the dark and also very limited directional swimming in the light when not exposed to a directional light source.
Discussion
Our results revealed strong negative effects of elevated CO2 on embryonic development and egg loss. There were no apparent effects on the more conventional measures of spawning and clutch sizes, though, our exposure time to the treatments were very short before spawning. Unlike most other studies of CO2 effects on wild animals, our study established an environment where natural reproductive behaviors could take place (spawning and embryo development during parental care), adding to the ecological relevance of the results. While embryonic malformations were likely a direct effect of elevated CO2, the cause of egg loss may have multiple causes. Possible explanations include that the parental male removed (ate) embryos not developing normally, or that elevated CO2 resulted in aberrant parental care behavior. Our results are in contrast to some studies on fishes reporting no negative effects of elevated CO2 on egg development (Munday et al. 2009a; Franke and Clemmesen 2011), but in line with recent findings for the temperate Atlantic fish Menidia beryllina for which elevated CO2 caused considerable mortality on the egg stage and a higher percentage of malformations in newly hatched larvae (Baumann et al. 2012). This suggests that early life stages can be negatively impacted by elevated CO2, but also that effects may vary among species. Marine fish larvae face a very high mortality, and mortality rates during larval and early juvenile stages significantly affect recruitment (Cushing 1990; Houde 1997). However, the potential effects of elevated CO2 on the population level remain to be investigated.
Experimentally elevated CO2 conditions caused a substantially stronger phototactic response in newly hatched larvae. This was not a general activity effect, but a specific effect on the response to a light stimulus, as larvae exposed to uniform overhead illumination showed a low level activity, which was not different between rearing treatments. In the dark almost no activity was detected. Moreover, we did not find any support for an acute effect of CO2 concentration of the test water. Thus, phototaxis was affected by CO2 conditions during embryonic development.
Positive phototaxis is generally considered to confer fitness benefits to fish larvae (Kamler 1992; Karlsen and Mangor-Jensen 2001). However, the effect of increased CO2 on the phototactic response in our study was very strong, with a 65–70 per cent increase in two different measures. Such a strong response may suggest visual hypersensitivity caused by elevated CO2, in line with the idea that high CO2 causes neuronal overactivity due to effects on brain neurotransmitter function (Nilsson et al. 2012). Visual sensitivity in animals is likely under stabilizing selection, with low sensitivity leading to an absence of an appropriate response, and hypersensitivity leading to maladaptive responses to weak or absent signals (Bradbury and Vehrencamp 2011). This is the first time a CO2 effect on phototaxis is tested. Further investigations are needed to establish whether the dramatic increase in phototactic response has positive or negative fitness effects for the larvae, and what the potential implications on the population level are. However, it is not unlikely that visual hypersensitivity is costly, as hypersensitive larvae may maladaptively respond to the many weak and accidental light stimuli in the complex visual environment of rocky shores kelp forests. Given that swimming toward the light was not monotonous, but interrupted by more or less frequent stops and turns by the larvae, we suggest that the increased phototactic response was likely caused by an increased sensory drive and a more directional and continuous swimming.
Larval phototaxis was affected by CO2 conditions during embryonic development but not by whether the test was conducted in elevated CO2 versus control water. This is consistent with previous work suggesting that short-term elevations of CO2 do not cause behavioral effects in coral reef fish larvae (Munday et al. 2010; Nilsson et al. 2012). Together with the present study, these works suggest that chronically elevated CO2 concentrations in a future ocean acidification scenario can be much more serious for fish than the sometimes large but short-term natural fluctuations that fish in many habitats (e.g., temperate coastal) experience today (Hofmann et al. 2011). When it comes to long-term effects of elevated CO2, a crucial question is whether organisms over multiple generations may adapt by selection of more tolerant genotypes and/or acclimate to climate stressors. It remains to be seen whether organisms with relatively long generation times, like fishes, have the potential to evolutionarily adapt to the rapidly increasing ambient CO2 levels. Results from tropical reef fish suggest that nongenetic parental effects may allow some acclimation to elevated CO2 over generations (Miller et al. 2012), but the long-term potential and generality of any such effect remains to be investigated.
In summary, the study reveals clear effects of elevated CO2 on embryos and larvae of a temperate goby. Increased CO2 resulted in higher egg loss and a higher incidence of abnormalities among developing embryos, in line with the prediction that negative effects should be evident at early life stages. We identified effects of elevated CO2 on vision-related behavior (phototactic response), as predicted by the hypothesis that CO2 affects sensory responses generally (see Munday et al. 2012). Specifically, newly hatched larvae reared under elevated CO2 conditions showed a dramatically increased phototactic response, in line with the recent finding by Nilsson et al. (2012) that high CO2 causes neuronal “overactivity”. Together with other recent findings (e.g., Baumann et al. 2012; Munday et al. 2012; Jutfelt et al. 2013), our results suggest that marine fishes are not as tolerant to increasing CO2 as previously thought. Notably, the effects of CO2 on sensory-related fish behavior seem not to be restricted to tropical reef fishes but may instead be of global concern.
March 2014